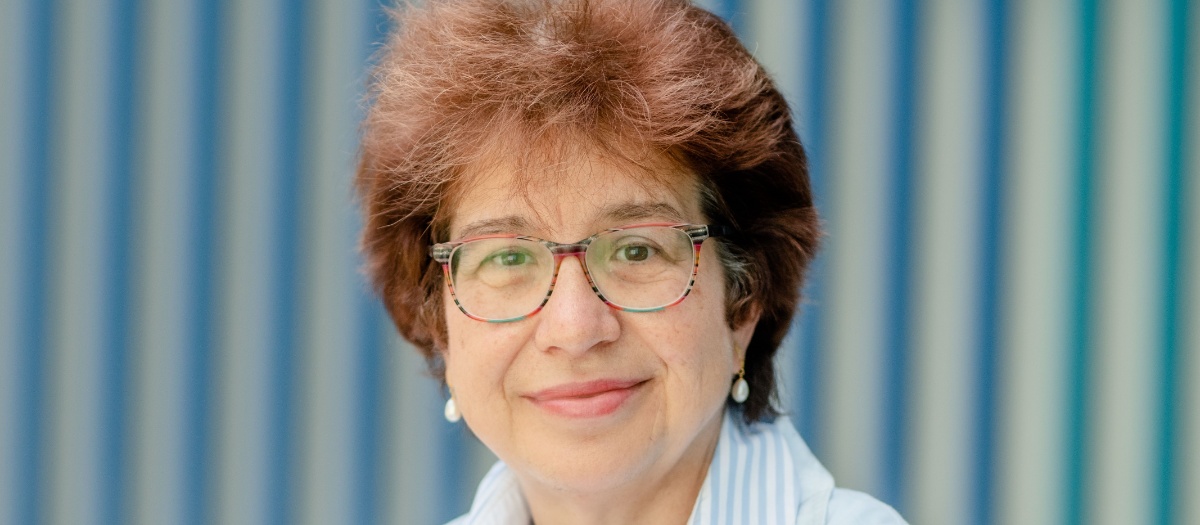
Exploring the unique role of water for life is one of the top future challenges in chemistry. Whether fast protein motion and solvent dynamics are correlated at the very heart of enzymatic reactions is still under heated debate. The underlying molecular mechanism of enthalpy-entropy (H/S) compensation in protein-ligand binding remains controversial. Systematic studies under steady state conditions revealed that differences in the structure and thermodynamic properties of the waters surrounding the bound ligands are an important contributor to the observed H/S compensation. These hydration free energies are dictated by a subtle balance of hydrophobic and hydrophilic interactions.
Calorimetry served as a powerful biophysical tool by measuring changes in thermodynamic state variables, but it is restricted to measurements in equilibrium and in macroscopic samples. THz calorimetry is a novel spectroscopic approach, which allows to deduce hydration free entropy and enthalpy based on spectroscopic observables. Thereby we can probe reach time resolutions of up to picoseconds and probe inhomogeneous samples. By probing the low frequency range which is most sensitive to the intermolecular interactions (100–600 cm−1), we established a direct correlation between spectroscopic observables and local contributions to the solvation free energy. In the talk I will outline the principles of THz calorimetry, as developed in my group, and present two applications:
Liquid–liquid phase separation (LLPS) describes the reversible compartmentalization of protein solutions into a protein-rich and a dilute phase; the former one being a local hotspot for neurotoxic aggregation in case of Alzheimer or Huntington disease. Using THz calorimetry, we observe how LLPS can be tuned by changes in hydration entropy and enthalpy. This allows to unravel two underlying molecular mechanism, which drive this process: The release of “Cavity-wrap” water hydrating hydrophobic patches during LLPS yielding an increase in entropy. In contrast, “Bound” water hydrating hydrophilic patches is retained. This process is enthalpically favorable. Both contributions favor protein aggregation in liquid droplets. A fundamental understanding of these processes is a prerequisite for tuning of LLPS.
The solvated electron is a fundamental reaction intermediate in physical, chemical, and biological processes. Using time resolved THz calorimetry we were able to follow the birth and time evolution of the solvated electron and compare these results directly to molecular simulations. We were able to observe in real time the evolution from a spectroscopic signature attributed to the delocalized electron (restricted to 500 fs) up to the spectroscopic signature of the localized electron, which is long lasting (>250 ps).
References:
1.
S. Pezzotti, B. König, S. Ramos, G. Schwaab, M. Havenith, Liquid–liquid phase separation? Ask the water!, J. Phys. Chem. Lett. 14, 1556–1563 (2023)
2.
F. Novelli, K. Chen, A. Buchmann, T. Ockelmann, C. Hoberg, T. Head-Gordon, M. Havenith, The birth and evolution of solvated electrons in the water, PNAS 120, e2216480120 (2023).
3.
S. Pezzotti, F. Sebastiani, E.P. van Dam, S. Ramos, V. Conti Nibali, G. Schwaab, M. Havenith, Spectroscopic fingerprints of cavity formation and solute insertion as a measure of hydration entropic loss and enthalpic gain, Angew. Chem. Int. Ed. 61, e202203893 (2022).